From Claire—If you missed it, here’s the first part of today’s Cosmopolicast: Energy Week: Beyond Thunderdome. Today we begin in medias res, so be sure to listen to them in order; otherwise, you’ll have no idea who’s who or why we’re all so agitated.
Energy Week has expanded well beyond the original plan; we’re now nearing on an Energy Month with the debate nowhere near settled, our readers hurling invective at us, and the Cosmopolitan Globalists barely on speaking terms. We’ve been calling each other Nazis on Twitter; one of us even suggested the other suffers from insecurity about his—well, frankly, never mind. Who knew? I sure didn’t when I suggested this debate. The good news is that we’ve discovered our acrimony is combustible, renewable, and more powerful than any of us imagined, so all we need to do is figure out how to harness it. Then we’ll be able safely to power the whole world, as well as Mars, well into the twenty-fourth century.
Frankly, though, we’re sick of the bickering. So until we all chill out, the Cosmopolitan Globalist will be sticking to bland, uncontroversial topics such as the Arab-Israeli conflict and whether it’s appropriate to encourage your transgender two-year-old to transition.
But before that, we’ve got to wrap things up. So today, on a hopeful note, Owen Lewis describes the promise of fusion. Tomorrow, of course, will be consecrated to Beautiful Things. On Monday, Dr. X will offer a last word about climate science, since he promised to try to answer all your questions. He won’t be able to answer every last one of them, but he has some thoughts about the best strategy, scientifically and epistemologically, for answering questions these kinds of questions.
Vivek has a terrific essay for us in the works about the economics of the energy transition, but we may wait until after the debate to publish it. There are other things happening in India right now that even more urgently require Vivek’s attention.
On Tuesday, we’ll award the Grand Prize to the best debater, and it will be very grand indeed. So hold onto your hats, because the winner will be feted in true Cosmopolitan Globalist style.1
How close are we to fusion power?
by Owen Lewis
Since the first laboratory demonstration of a fusion reaction in the early 1930s, scientists have been trying to figure out how to harness the kind of energy that powers the Sun and the stars. A fusion device that produces more power than required to sustain the reaction—a result called net energy—is the holy grail. The power would be clean, reliable, and inexhaustible. It would generate significantly more power per kilogram of fuel than any other source in our grasp, including nuclear fission. What’s more, it wouldn’t emit a drop of particulate matter, carbon dioxide, or any other noxious byproduct. So far, no one has managed it. But we’re closer than you might think.
Nuclear fusion and nuclear fission are significantly different. Both processes involve atoms, and both convert mass into energy, as described in Einstein’s famous equation, E=mc2. But to achieve nuclear fission, you bombard a large atom, like uranium, with high-speed particles to split it into smaller atoms, unleashing an enormous amount of energy in the process. To achieve nuclear fusion, however, you go the opposite direction: It occurs when two small atoms, like hydrogen, are fused into a larger one—again releasing significant energy.
It’s not quite that simple, of course. Almost all fusion in the Sun takes place as a result of what’s known as a proton-proton chain reaction. This begins with hydrogen. Hydrogen atoms are the most abundant in the universe and the simplest: just one proton and one electron. Take away the electron, and a hydrogen atom is a naked proton. The Sun’s searing heat ionizes hydrogen into protons, then the far more immense heat and pressure at the Sun’s core fuses them into helium, the second most common atom in the universe. In the process, a small amount of the mass of the original protons, just shy of one percent, is released as energy.
It would be impractical to replicate this on the Earth. So fusion reactors here won’t begin with hydrogen, but with its isotopes. Atoms are isotopes if they have the same number of protons in their nucleus but a different number of neutrons. Hydrogen has three isotopes, protium (usually just called hydrogen), deuterium, and tritium. Many planned fusion reactors will run on deuterium-tritium fuel, because it’s comparatively easy to work with; it fuses at a mere 150 million degrees K—only ten times hotter than our Sun’s core.
Deuterium is abundant. It can be extracted from seawater. Tritium is trickier. It’s extremely rare in nature. Indeed, our current supply of tritium is an estimated twenty kilos, all artificially produced in nuclear fission reactors. What’s more, it’s radioactive. Reliance upon tritium would thus pose “formidable technology and sustainability challenges,” in the words of a laboratory working on a competitive process. But we may be able to breed it: Researchers are hard at work now trying to figure out whether tritium can be coaxed out of the interaction of the neutrons produced by fusion and a lithium blanket around the reactor.
The problem with D-T is that some 80 percent of the energy in the reaction takes the form of high-energy neutrons. Unfortunately, these slowly turn the materials with which they interact radioactive, so parts of the reactor need to be replaced every few years. But the radioactivity, both from the tritium and from bombardment by high energy neutrons, is mild. The parts need only be stored a few decades, unlike the waste from fission reactors, which must be stored for centuries or millennia. It needs to be handled with care, but it’s not inherently more dangerous than the many forms of medical and chemical waste that modern societies handle safely and routinely.
D-T isn’t the only possible fuel, though other candidates seem less plausible, given the higher temperatures they require. Most private companies favor D-T, as do big government projects like the International Thermonuclear Experimental Reactor. But a few researchers are working on other ideas. So far, the most promising rival is hydrogen-boron plasma—the product of a fusion reaction involving a proton and a boron-11 nucleus, or P-B11. The goal is ignition—a reaction that produces enough power to maintain its own temperature without heat from another source. Hydrogen-boron plasma would have to be at least ten times as hot as deuterium-tritium plasma for ignition, so some laboratories are trying to induce the plasma to fuse with short bursts of energy from high-powered laser beams. This strategy has the advantage of being aneutronic, meaning it doesn’t produce neutrons as waste at all, just charged products that can be directly put to use making electricity.
There are many reactor designs, but the most tested are the tokamak (an acronym for the Russian words for toroidal magnetic confinement) and the stellarator. Both are toroid-shaped. (A donut is a toroid. So is a coffee cup. But the image you want here is your classic donut.) Both use magnets to confine and stabilize the plasma as it fuses. These days the majority of large government projects, and several private ones, are tokamaks, but stellarators are coming back in vogue.
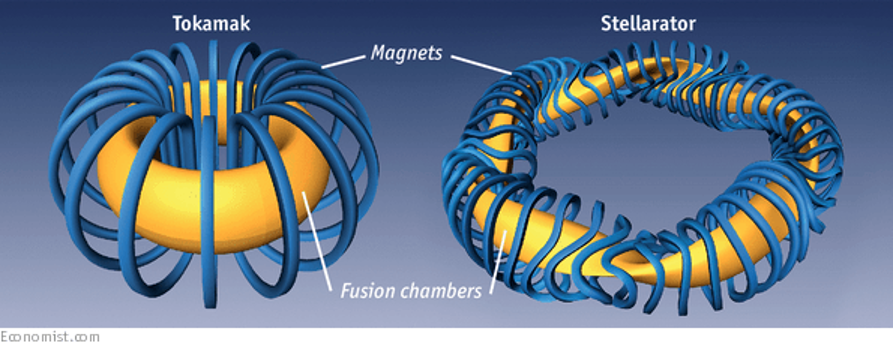
The International Thermonuclear Experimental Reactor, the world’s largest fusion project, broke ground in the south of France in 2007. Researchers at ITER, as it’s known, aim to make it a proving ground for fusion technology: They aim to produce a net energy gain of 500 thermal megawatts from a 50 megawatt input. ITER won’t actually produce electricity: It’s meant to be a precursor to an even larger tokamak, the Demonstration Power Plant, or DEMO, which would prove that nuclear fusion can indeed produce electricity—inspiring the design of more efficient and less costly power plants.
The problem with ITER is the timeline and the cost. The first plasma tests won’t start until 2025; D-T fusion won’t begin until 2035. DEMO operations won’t begin until the mid-2050s. The project has been plagued by delays and cost overruns. The original budget of US$7 billion has ballooned to US$24 billion, making it the world’s second-most expensive scientific endeavor after the International Space Station.
On their website, ITER claims they’ll produce the first fusion device to produce net energy. But they’re apt to be beaten. A number of governments have decided they can’t wait another thirty-odd years for ITER to prove itself and have begun their own programs, with accelerated timelines. Earlier this year, the US Department of Energy’s Fusion Energy Sciences Advisory Committee and the US National Academies of Sciences, Engineering, and Medicine each published reports calling for massive public and private investment in an American fusion power plant, with the goal of providing electricity to the grid between 2035 and 2040. A bipartisan fusion caucus in Congress formed at the same time. Meanwhile, the UK has set aside £220 million (US$311 million) for the Spherical Tokamak for Energy Production, or STEP. They aim to build a prototype plant that produces electricity by 2040.
Nor are Western governments the only ones on the fusion quest. China is designing the China Fusion Engineering Test Reactor. The two-phase plan recalls ITER and DEMO, but their timeline is faster; they aim for completion of the first phase in the 2030s and the second in the 2040s.
Progress in the private sector has been encouraging, too. At last count, thirty companies had together raised some US$2.5 billion in investments. It’s a paltry sum compared to private investments in renewable energy, but it’s a huge increase compared to next-to-nothing, which was where we were a decade ago. In 2018, private companies formed the Fusion Industry Association to speak for the nascent industry. It has already begun to work with governments to develop appropriate industry regulations.
No clear industry leader has yet emerged, but the California-based TAE Technologies has raised the most money. They plan to start with D-T fuel, but their goal is aneutronic fusion using P-B11. Commonwealth Fusion Systems in the United States and Tokamak Energy in the UK are both working on compact spherical tokamak reactors—shaped more like a cored apple than a donut. Advances in high-temperature superconductors will help them both to build smaller, more powerful, and ultimately less expensive devices. Canada’s General Fusion is another promising start up; they’re using multiple pistons to compress a liquid metal shell; this in turn compresses plasma to fusion conditions. All of these companies believe their Kitty Hawk moment is coming in this decade; they aim to start providing electricity by 2030. It remains to be seen whether they’re right, but recent, peer-reviewed papers published in The Journal of Plasma Physics suggest the Commonwealth Fusion’s SPARC tokamak is indeed headed toward net energy.
Smaller companies are vying for a share of the pie, too. Australia’s HB11 Energy is pursuing aneutronic fusion through an innovative, laser-based method that might be far simpler and more effective than other approaches. It’s early days, but the company has raised several million dollars.
Why are we seeing this fusion renaissance? Technological advances have certainly played a role, particularly better computers with their plasma modeling power, and high-temperature superconductors that allow for stronger magnetic fields and better plasma confinement. These will ultimately lead to cheaper machines. But the biggest change has been funding. Apart from ITER, fusion research and development had been on a starvation budget for decades. Indeed, it’s remarkable that anyone made much progress at all.
Programs such as the Advanced Research Projects Agency–Energy in the United States have provided vital starter funds for research. In some cases, this led to further private investment. The program remains woefully underfunded, but fusion advocates hope the Biden Administration will increase spending in this area. If the US is serious about building their own fusion power plant, they will have to get equally serious about ramping up funding.
Let’s say we succeed in building fusion power plants. Will they be able to produce enough cheap electricity to compete with other sources? We can’t know for sure because we just haven’t got any working reactors yet. But we can be cautiously optimistic. Nicholas Hawker, the CEO of First Light Fusion, thinks that once the technology is mature, his company’s approach could deliver a levelized cost of energy as low as US$25/MWh. That would be much cheaper than nuclear energy, at about US$100/MWh. It would be half the price of onshore wind.
Even if it costs more than this, the fuel itself is essentially inexhaustible and its price insignificant. The power plants would be safe. Their impact on the environment would be minimal. They would also provide power that’s always on and reduce the need for expensive infrastructure investments such as long distance transmission lines. So fusion-generated electricity could be very competitive.
Nuclear fusion power, though, is about so much more than clean energy. With abundant fusion power we could end energy poverty once and for all, globally—and thus end poverty, period.
Nor should we keep our aspirations confined to Earth. Fusion rockets and compact power plants could allow us to expand into and past our Solar System, faster and further than otherwise possible. Soon we could be zipping from Earth to Mars, or out to the asteroid belt, in fusion-driven spaceships. The only way to travel to nearby stars within a human lifetime, probably, is on a fusion rocket. As Robert Zubrin wrote in The Case for Space,
That is a promise not to be taken lightly. It is the reason controlled fusion must be pursued despite all apparent obstacles, setbacks, and attractive alternatives. The stars are worth more than kilowatts.
There’s a lot of work to do, but fusion energy is now a question of when, not if. And the when could be here a lot faster than most people realize.
Owen Lewis (@is_fusion) is a science and space enthusiast and Christian humanist with degrees in geology and psychology. He is the Founder of Fusion is the Future.
Special note to Alan Potkin: We’re going to hold your thoughts about Myanmar until after the debate. We think they stand on their own. Also, we don’t rightly know if our readers are heartily sick of Energy Week, but we figure it’s time to wrap this exercise up while some of the editors, at least, are still speaking to each other.
Share this post